Icy Super-Earths: Insights from Microlensing Surveys
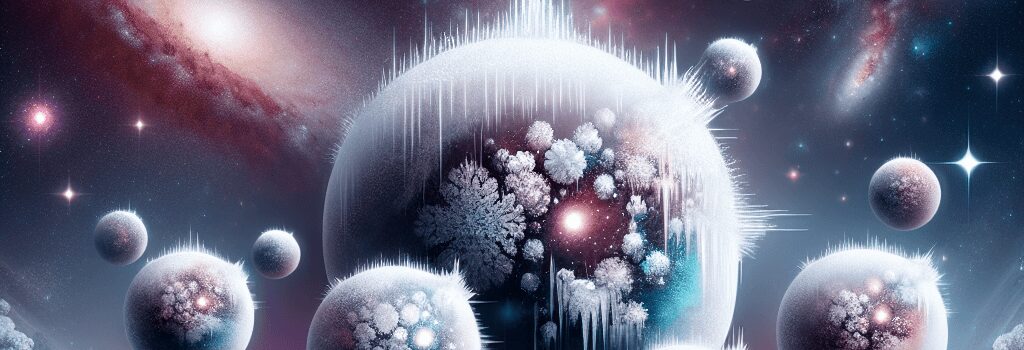
In a groundbreaking study published in Science (DOI: 10.1126/science.adn6088), researchers from the Korea Microlensing Telescope Network (KMTNet) have unveiled compelling evidence that our Galaxy hosts a large population of rocky “super-Earths” on distant, icy orbits. By exploiting the gravitational microlensing technique, which is sensitive to planets at separations of several astronomical units (AU), the team has begun to fill a critical gap in our understanding of exoplanet demographics beyond the reach of transit and radial-velocity surveys.
Context and Motivation
Most of the ~5,000 confirmed exoplanets orbit within 1 AU of their host stars, a bias imposed by methods that favor short orbital periods. In contrast, cold planets—like those residing near Jupiter’s or Saturn’s orbital distances—remain largely uncharted territory. Microlensing events occur when a foreground star (the “lens”) and any accompanying planet align closely with a background source star, briefly magnifying its light. These rare events offer a unique window onto planets in wide orbits, including those that never transit and those whose gravitational tug on the host is too small for radial-velocity detection.
Gravitational Microlensing: Principles and Implementation
Gravitational microlensing relies on Einstein’s general relativity: a lens mass M at distance D_L from Earth and D_S from the source produces an Einstein radius R_E = sqrt(4GM D_L(D_S–D_L)/(c^2 D_S)). For a 0.3 M☉ lens at 4 kpc, R_E ≈2 AU. KMTNet’s three 1.6 m f/4 telescopes—located in Chile, South Africa, and Australia—are each equipped with 18k×18k CCDs (0.4″/pixel, 4 deg² field) observing primarily in I-band with 2–5 min cadence. Real-time anomaly detection pipelines use convolutional neural networks to flag planetary deviations within minutes of occurrence.
Case Study: OGLE-2016-BLG-0007L
The event OGLE-2016-BLG-0007, first reported by the Optical Gravitational Lensing Experiment (OGLE), displayed a primary magnification lasting ~120 days with a short 8-hour anomaly. Detailed modeling using inverse ray-shooting and Markov Chain Monte Carlo fitting yields a mass ratio q≈2×10⁻⁵. Assuming a host mass M_L≈0.3 M☉ (typical mid-M dwarf), the planet mass M_p≈1.3 M⊕. The projected separation a⊥≈5 AU implies a true semi-major axis a≈10 AU—comparable to Saturn’s orbit, but with a predominantly rocky composition.
Population Statistics and Planet Formation Implications
Analyzing over 60 KMTNet detections with a hierarchical Bayesian framework that corrects for detection efficiency as a function of q and normalized separation s, the study reveals two distinct populations: (1) super-Earths (1–10 M⊕) at s≈1–5 (a≈1–10 AU) and (2) Neptune- to Jupiter-mass giants (10–300 M⊕) at s≈1–4. This dual-peaked distribution aligns with core-accretion theory: planetary cores grow to a critical mass (~10 M⊕) before run-away gas accretion, separating icy super-Earths from gas giants.
Technical Challenges and Instrumentation
Microlensing surveys contend with finite-source effects, photometric systematics, and stellar blending in the Galactic bulge. KMTNet’s longitudinal coverage mitigates diurnal gaps, while difference image analysis (DIA) achieves ~1% photometric precision at I=17 mag. Dr. David Bennett (University of Maryland) notes, “Future surveys must integrate adaptive optics follow-up to resolve lens flux, breaking the mass–distance degeneracy inherent to microlensing.”
Expert Opinions and Theoretical Context
Prof. Andrew Gould (Ohio State University) comments, “This work refines our view of the mass function beyond the snow line, showing the Galaxy teems with dormant, icy super-Earths.” Dr. Jane Rigby (NASA Exoplanet Exploration Program) adds, “Combining microlensing discoveries with JWST and the Roman Space Telescope direct imaging will soon enable atmospheric studies of these cold worlds.”
Future Prospects and Next-Generation Surveys
NASA’s Roman Space Telescope (launch 2026) will perform a high-latitude microlensing survey with a 2.4 m aperture and 0.28 deg² infrared field, offering 15-min cadence sensitivity to Earth-mass planets at 1–5 AU. Roman is expected to detect ≥100 cold terrestrial planets. Complementary programs from ESA’s Euclid mission and the Vera C. Rubin Observatory’s LSST will extend sensitivity to free-floating planets and the low-mass tail of the mass function.
Conclusions
These expanded microlensing results reveal that Solar-System-style architectures—lacking distant icy super-Earths—may be the exception rather than the norm. The observed dual mass distribution supports classical core-accretion models, while forthcoming facilities promise to complete the census of cold exoplanets. As instrumentation and analysis techniques evolve, we are poised to enter a golden era of discovery at the outer reaches of planetary systems.