Falcon 9 Sonic Booms: Seismic Wave Analysis
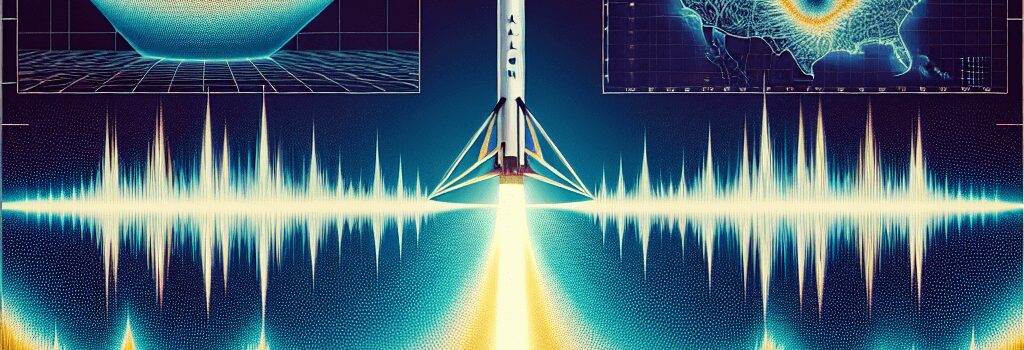
When SpaceX’s Falcon 9 boosters accelerate beyond Mach 1, the resulting sonic booms don’t just crack the sky—they can reverberate through the ground like seismic waves. While a sonic boom is typically experienced as a sharp popping noise, under certain conditions it can be felt as a low‐frequency rumble, raising concerns for communities near the launch trajectory. This article explores the physics behind these phenomena, the environmental factors that shape them, and the latest observational data.
1. Shockwave Generation and Propagation
Falcon 9’s nine Merlin 1D+ engines produce a combined thrust of nearly 8 MN at sea level. As the vehicle climbs through the atmosphere, its velocity transitions from subsonic to supersonic, generating overlapping Mach cones—conical shockwave fronts that coalesce into a sonic boom.
- Overpressure pulses typically range from 20 to 200 Pa (0.003–0.03 psi).
- Pulse durations are on the order of 0.1–0.5 s, depending on altitude and speed.
2. Influencing Factors
2.1 Trajectory and Mach Cone Geometry
The angle of the Mach cone (μ) is given by sin⁻¹(1/M), where M is the Mach number. As Falcon 9 accelerates from Mach 1 to Mach 2.5, μ narrows from 90° to around 23°, concentrating acoustic energy in a tighter footprint on the ground.
2.2 Wind Shear and Atmospheric Layers
Wind shear—changes in wind speed and direction with altitude—bends shockwave paths through refraction. On launch days with strong upper‐level westerlies and calmer surface winds, local residents downrange may report a prolonged rumble lasting up to 30 seconds.
2.3 Temperature Gradients and Refraction
Temperature inversions can trap acoustic energy in a ducted layer, akin to atmospheric waveguides. During a recent Transporter‐9 mission, ground sensors recorded a 40% boost in overpressure when a strong inversion at 1,200 m refracted shockwaves back toward the surface.
3. Topographical and Surface Effects
Coastal bluffs, canyons, and even large buildings can reflect and focus shockwaves, similar to how seismic waves interact with geological faults. In some areas near Cape Canaveral, residents have reported vibrations comparable to a magnitude 2.0 micro‐earthquake.
4. Acoustic Propagation Modeling
Advanced Computational Fluid Dynamics (CFD) and Parabolic Equation (PE) models are now used to forecast sonic boom footprints. By incorporating real‐time radiosonde data and LIDAR wind profiles, these models can predict overpressure contours within ±5 Pa accuracy up to 200 km downrange.
5. Mitigation Techniques and Regulatory Requirements
- Flight‐Path Optimization: Adjusting the pitch‐over program to modulate altitude and speed, reducing overpressure at sensitive zones.
- Engine Throttling: Temporarily throttling Merlin engines during transonic flight to smooth shockwave formation.
- Community Alerts: Notifying local populations via automated sirens and mobile alerts when predicted overpressures exceed 50 Pa.
6. Case Study: Coastal Launch Observations
“Sensors deployed along Florida’s Space Coast recorded a ground acceleration of 0.02 g during the June 2024 launch, correlating to a 120 Pa overpressure spike,” explains Dr. Elena Morales, an aerospace acoustics specialist at the University of Central Florida.
7. Environmental Impact and Future Outlook
Repeated low‐level rumbling can affect wildlife sensitive to infrasound, such as bats and cetaceans. SpaceX and NASA are collaborating on environmental impact studies, deploying seismometers and infrasound arrays to refine their mitigation strategies.
As launch cadence increases with Starlink missions and NASA contracts, understanding and controlling sonic booms will be vital to sustainable space operations.