Airflow-Powered Soft Robot Mimics Lifelike Motion
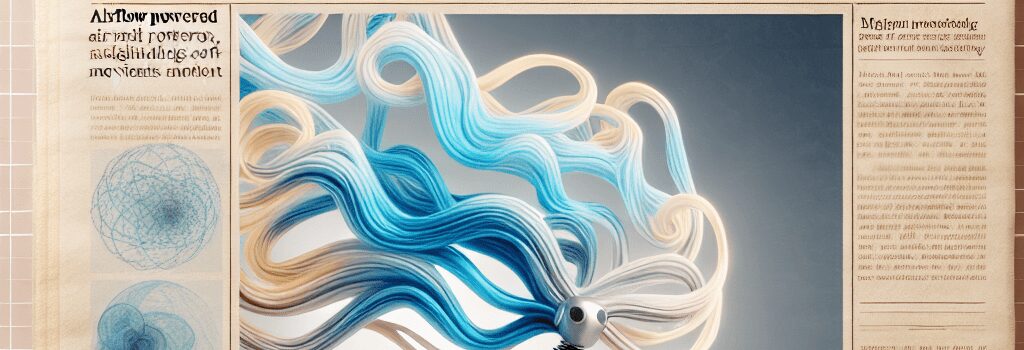
Traditional robots depend on central processors, heavy sensor suites, and AI algorithms to coordinate movement—resulting in bulky designs and often stilted gaits. In contrast, a team at the FOM Institute for Molecular and Atomic Physics (AMOLF) in Amsterdam has unveiled a radically simple, brain-free soft robot that uses nothing more than the physics of airflow and elastic tubing to run, vault obstacles, and even swim.
Sky-Dancing Physics: From Laboratory Curiosities to Autonomous Motion
The story began when roboticist Alberto Comoretto bent a pneumatic tube to stop airflow. Instead of remaining still, the tube entered a self-sustaining oscillation at 120–200 Hz, producing a loud whistle. High-speed video (2,000 fps) revealed that kinks formed at constriction points, propagated along the 50 mm-diameter, 1.5 mm-thick silicone tube, released pressure, and then reformed—creating a periodic, asymmetric waveform.
“We recognized the same principles behind the 1996 Olympic ‘Fly Guys,’” says Comoretto, referring to the dancing inflatable figures powered by alternating air pulses. “But their motion was random. We wanted controlled periodicity, mimicking how muscles and tendons drive living organisms.”
Tubular Legs: Geometry-Driven Gaits
By 3D-printing a 120 mm-long soft chassis and attaching four bent pneumatic legs, the team programmed gait frequencies via inlet pressure. Air pressures in the range of 15–30 kPa generated oscillations at 80–150 Hz. Remarkably, limb synchronization emerged without electronic coordination—akin to metronomes syncing on a floating board.
Key specifications:
- Leg tube material: silicone elastomer (shore hardness 30A)
- Tube inner diameter: 5 mm; wall thickness: 1.5 mm
- Operating pressure: 10–40 kPa
- Oscillation frequency: 50–200 Hz
- Pneumatic coupling: single manifold input
On hard surfaces, all four legs flapped in unison—producing a stotting gazelle gait at up to 0.2 m/s. Submerged in water, reduced drag caused limbs to fall into antiphase, resulting in a dog-paddle swim cycle.
Advanced Power and Efficiency Enhancements
Early prototypes required an 85 W laboratory pump delivering 15 L/min, tethered via a 3 m hose—a serious barrier to autonomy. To address this, the team:
- Reduced the limb count from four to two, halving airflow demand
- Refined tube curvature and wall thickness to lower kink-initiation pressure to 8 kPa
- Integrated a µ-compressor (0.5 g/cm³, 7 kPa peak) drawing 120 mA at 7.4 V
The result: 0.06 W per limb and a 250 mAh Li-ion battery supporting 20 minutes of untethered operation. The new robot weighs 35 g and measures 100 mm × 40 mm × 30 mm.
Physical Intelligence and Emergent Coordination
Without microcontrollers, the robot relies on physical intelligence—where body–environment interactions perform the ‘computation.’ Pressure waves within the shared manifold couple the limbs, allowing them to adapt gait in real time. When one limb encounters an obstacle or fluid resistance, pressure feedback automatically alters oscillation timing across all actuators.
Dr. Jane Liu, a soft robotics expert at MIT, notes: “This is a significant step toward fully embodied intelligence. The robot’s morphology does the signal processing that would otherwise require software.”
Emergent Control Strategies with Minimal Electronics
To steer behavior, the team added two photodiodes as simple “eyes”. Analog comparators trigger pneumatic valves that open or close each limb’s inlet port based on ambient light thresholds. As a result, the robot can:
- Navigate toward a light source
- Switch from running to swimming upon water detection (via capacitance sensing)
- Avoid walls by differential limb activation
“Currently, collisions make it veer left,” explains co-author Johannes Overvelde. “We’re characterizing the nonlinear dynamics so we can tune responses for specific tasks.”
Potential Applications and Future Directions
With no complex software stack, such soft robots could excel where robustness and adaptability are paramount:
- Biomedical devices: artificial hearts or peristaltic pumps that self-adjust to blood pressure fluctuations
- Environmental monitoring: lightweight swimmers for water quality sensing in fragile ecosystems
- Search and rescue: air-driven crawlers that squeeze through rubble without electronics prone to failure
Looking ahead, the AMOLF team is collaborating with EPFL to integrate shape-memory polymers for variable stiffness and with TU Delft to embed biodegradable tubing for single-use medical applications. A paper detailing the full pneumatic circuit design and nonlinear modeling appears in Science (2025, DOI: 10.1126/science.adr3661).
Expert Perspectives
Prof. Maria Vasquez, mechanical engineer at Caltech, comments: “This work exemplifies the shift from digital control to physical computing—where intelligence is ‘written’ in material properties and geometry. The challenge now is scaling complexity without losing reliability.”
Conclusion
By harnessing the intrinsic dynamics of airflow and soft materials, the AMOLF team has charted a path to robots that are simpler, lighter, and more adaptable than their digitally controlled counterparts. As research into embodied intelligence advances, we may soon see a new generation of machines whose ‘brains’ are in their bodies and environments—no software updates required.