Commercial Fusion Power Advances: Test Systems Pave the Way for a Clean Energy Future
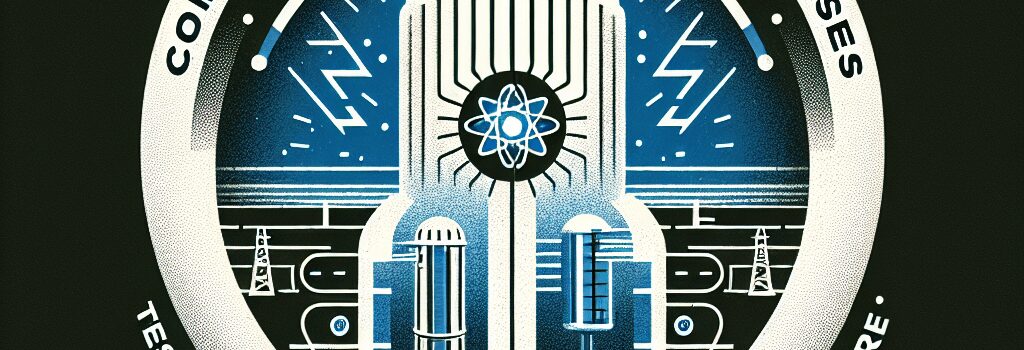
The Evolving Landscape of Fusion Technology
Commercial fusion power startups are rapidly emerging, transforming a system once thought to be perpetually out of reach into a tangible near-future reality. Companies are pursuing radically innovative solutions for heating and compressing plasmas or fusing unusual combinations of isotopes, while also embracing tried-and-true designs that have undergone decades of academic scrutiny. The fusion sector now finds a dynamic balance between cutting-edge innovations and established physics, leading to impressive advances in test systems and prototype reactors.
Tokamak Developments: The SPARC Milestone
One of the most promising efforts comes from Commonwealth Fusion, which is building a demonstration tokamak known as SPARC in Massachusetts. A tokamak—a donut-shaped chamber that uses intense magnetic fields to control plasma—remains one of the most studied and promising designs in fusion energy. SPARC aims to leverage high-temperature superconductors to achieve ITER‐level performance in a significantly smaller and more efficient package. Recently, Commonwealth Fusion reached a critical milestone with the installation of the first tokamak component: the cryostat base.
The cryostat base is in charge of maintaining cryogenic temperatures for the superconducting magnets. Engineer Alex Creely explained that its materials must withstand extreme conditions—not only operating at temperatures around 20 Kelvin but also resisting the neutron bombardment inherent to fusion reactions. Stainless steel, with its robust thermal properties and neutron tolerance, ensures that the structure can handle an extreme temperature gradient: a transition from the plasma’s hundreds of millions of degrees Celsius to around 1,000°C within a 30-centimeter span before reaching the cryogenic environment. This engineering feat highlights the intricate nexus of material science, thermal dynamics, and magnetic field control that underpins modern tokamak operations.
Stellarator Innovations and Advanced Design Strategies
In parallel with tokamak progress, several companies are pushing forward stellarator designs, which inherently offer different advantages. Stellarators create a complex three-dimensional magnetic configuration to sustain a stable, continuous plasma flow—a feature that makes them attractive as steady-state fusion reactors. Type One Energy, for instance, has advanced its stellarator concept with extensive peer-reviewed research, including six articles published in the Journal of Plasma Physics. Their work covers structural design, plasma behavior, helium handling, tritium generation through neutron interactions, and efficient heat extraction.
Thea Energy is also in the mix, emphasizing a simplified hardware approach. By replacing complex three-dimensional magnets with a flat array of high-temperature superconducting magnets, Thea Energy relies on sophisticated software to emulate the necessary magnetic fields. CEO Brian Berzin notes that this strategy could reduce manufacturing complexity and cost, allowing for the modular replacement of components during maintenance. In an inventive twist, Thea Energy plans to use deuterium-deuterium fusion for initial testing, incorporating a lithium layer that generates tritium upon neutron bombardment. Such innovations underline a broader trend of leveraging computational design and advanced materials to refine stellarator technology for future energy production.
Technical Analysis: High-Temperature Superconductors in Fusion Reactors
One of the technical linchpins in these reactors is high-temperature superconductivity. Unlike conventional superconductors that require extremely low temperatures to operate, high-temperature superconductors offer improved efficiency and stability under more accessible cryogenic conditions. They enable the creation of much stronger magnetic fields, which are necessary to confine plasma with high energy density. The significant reduction in cooling requirements not only lowers operational complexity but also enhances the overall economic viability of fusion reactor designs. This breakthrough in superconducting technology is fundamental to both the SPARC tokamak and the innovative stellarator designs currently under development.
Deeper Analysis: Economic and Energy Market Implications
While operational fusion power plants may still be years away, the move towards constructing test reactors signals a promising shift in the energy landscape. Economic analysts suggest that the gradual convergence of reliable fusion test systems with evolving renewable sources could redefine global energy markets. As fusion startups receive increasing backing from public and private sectors, the improved supply chain—bolstered by learnings from ITER—will likely decrease costs and accelerate the transition from experimental reactors to commercial systems. However, these developments must be seen in the context of broader market uncertainties, regulatory hurdles, and rapid advancements in alternative clean energy technologies.
Looking Forward: Expert Opinions and Industry Collaboration
Collaboration is a key driver in the field of fusion research. Experts highlight that the synergy between academic studies, such as those advancing ITER, and commercial ventures is highly beneficial. With partnerships already in place—Commonwealth Fusion is working with vendors that also supply components to ITER, and stellarator developers are collaborating with institutions like Oak Ridge National Lab and the Tennessee Valley Authority—these projects are laying the groundwork for a robust, scalable fusion industry.
Technical Specifications and Future Roadmap
- Construction Milestones: Completion of the cryostat base component is expected within a year, ushering in the final construction phase of the reactor test systems.
- Commissioning Schedules: Following construction, a dedicated commissioning period of approximately one year is planned, with initial fusion experiments slated for 2027.
- Materials and Design: Utilization of stainless steel to handle temperature gradients from above 100 million degrees Celsius down to cryogenic levels (around 20 Kelvin) underscores the engineering challenges overcome in current tokamak and stellarator designs.
- Magnet Systems: Transition from complex three-dimensional magnets to simplified arrays via advanced software algorithms, promising reduced cost and improved maintainability for future reactors.
In conclusion, although commercial fusion power plants may not be online for another decade, the progress made by innovators like Commonwealth Fusion, Type One Energy, and Thea Energy signifies a decisive breakthrough. With a focus on both incremental improvements and innovative leaps in design and materials, the fusion community is steadily moving from theoretical sketches to tangible, operational hardware. This transition is set to play a crucial role in the global shift towards sustainable and high-performance energy solutions in the coming decades.