Revolutionizing Healthcare: The Impact of 3D Printing
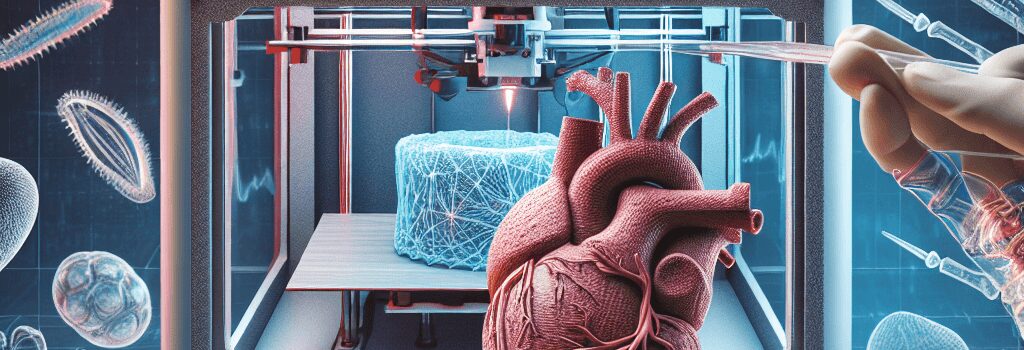
Three-dimensional (3D) printing is transforming medical care by shifting from mass-produced solutions to patient-specific treatments. From bespoke prosthetics to bioprinted tissues and on-demand pharmaceuticals, these technologies are enabling unprecedented customization.
Custom Prosthetics and Implants
Modern prosthetics leverage a variety of 3D printing modalities—stereolithography (SLA), selective laser sintering (SLS), fused deposition modeling (FDM), and digital light processing (DLP). Each offers unique resolutions (20–100 µm layer thickness) and material compatibilities:
- PEEK and Medical-Grade Nylon: High tensile strength (~90 MPa), biocompatibility, used for load-bearing implants like spinal cages.
- Photopolymer Resins: Ultra-fine resolution (<50 µm) for facial implants, dental crowns, and surgical guides.
- Bioinks and Hydrogels: Composite formulations of alginate, gelatin methacrylate (GelMA), and decellularized extracellular matrix for soft-tissue scaffolds.
“The integration of AI-driven topology optimization with high-resolution SLA has reduced implant weight by 30% while maintaining structural integrity,” says Dr. Jane Smith, Senior Biomaterials Scientist at BioFab Labs.
Advances in Bioprinting and Tissue Engineering
Bioprinting emerged in the early 2010s with inkjet and extrusion-based systems depositing living cells directly into 3D matrices. Key milestones include:
- 2013: Organovo’s first 3D-bioprinted liver tissue, demonstrating >80% cell viability post-print.
- 2024: University of Tokyo’s trial of bioprinted cartilage implants showing 95% integration rate in animal models.
- 2025: Northwestern University announced a pilot study on bioprinted kidney proximal tubule arrays for drug screening.
Optimizing nozzle diameters (100–200 µm), extrusion pressures (50–150 kPa), and bioink stiffness (1–10 kPa) remains critical to preserve cell function and promote vascularization.
Pharmaceutical Printing and Personalized Dosage
3D printing in pharma enables custom-dosage, multidrug tablets with complex release profiles. Spritam® (levetiracetam) was FDA-approved in 2015 using ZipDose® technology to achieve rapid dissolution of high-dose formulations. Recent developments include:
- Multi-Material Inkjet Printing: Deposits active APIs and excipients in microarrays for controlled release over hours to days.
- On-Site Pharmacy Integration: Trials at Massachusetts General Hospital utilize FDM-based drug printers to produce patient-specific regimens for pediatric oncology.
Simulating Surgeries with Anatomical Models
Surgeons now routinely use patient-derived CT/MRI data to 3D print anatomical replicas. Benefits include:
- Preoperative Planning: Reduces OR time by 15–25% and complication rates by 10% in complex cardiac and craniofacial surgeries.
- Real-Time Navigation: Integration with AR headsets allows overlaying 3D prints onto the patient intraoperatively.
Regulatory and Quality Assurance in 3D-Printed Medical Devices
Ensuring safety and consistency in 3D-printed medical products demands robust validation protocols:
- ISO 13485 certification for manufacturing systems.
- FDA’s Technical Considerations for Additive Manufactured Medical Devices guidance, focusing on material properties, process controls, and post-processing requirements.
- Real-time in-line monitoring using optical coherence tomography (OCT) and machine vision to detect layer defects.
AI-Driven Design and Digital Twins
Artificial intelligence is accelerating custom device development:
- Topology Optimization: AI algorithms analyze patient-specific load conditions to generate lightweight, mechanically optimized lattice structures.
- Digital Twins: Virtual replicas simulate long-term fatigue and wear, predicting failure modes before physical prototyping.
- Image Segmentation: Deep learning models automatically convert DICOM data into printable STL files in under 10 minutes.
Economic and Accessibility Considerations
While 3D printing can reduce per-unit costs by 40–60%, initial capital expenses for industrial-grade printers (USD 100,000–500,000) and certified materials pose barriers. Initiatives to widen access include:
- Open-Source Prosthetic Projects: e-NABLE community has provided over 10,000 free 3D-printed hands to underserved regions.
- Shared Service Bureaus: Regional health networks pool resources to offer low-cost printing as a service.
- Training Programs: Partnerships between universities and vocational schools to certify 3D printing technicians in ISO standards and biocompatibility testing.
Future Directions and Challenges
Key research frontiers include:
- 4D Printing: Smart polymers that change shape or stiffness in response to temperature or pH, enabling dynamic implants.
- In Situ Bioprinting: Techniques using ultrasound or microfluidic nozzles to print directly into wound beds, reducing surgical steps.
- Nanocomposite Resins: Embedding antimicrobial nanoparticles to prevent post-op infections.
“The convergence of materials science, AI, and advanced manufacturing heralds a new era of truly personalized medicine,” concludes Dr. Robert Lee, Chief Innovation Officer at MedPrint Solutions.